Introduction:
A solar cell (PV cell) is an electronic device that converts the energy of light directly into electricity by means of the photovoltaic effect. It’s essentially a semiconductor device that absorbs sunlight, exciting electrons, which then flow through an electrical circuit, generating electricity.
Solar cells are made from materials like silicon, which are specially designed to absorb sunlight and convert it into electrical energy. They’re typically arranged in a grid-like pattern on a solar panel, which can be used to power a wide range of devices, from small electronics to entire buildings and even grids.
History:
The history of solar cells dates back to the 19th century, with significant advancements in the 20th century. Here’s a brief overview:
- 1839: French physicist Edmond Becquerel discovers the photovoltaic effect, where light generates an electric current.
- 1873: Willoughby Smith discovers the photoconductivity of selenium, leading to the development of the first solar cells.
- 1883: Charles Fritts creates the first commercial solar cell using selenium, with an efficiency of around 1%.
- 1904: Albert Einstein explains the photoelectric effect, laying the theoretical foundation for solar cells.
- 1941: Russell Ohl patents the first modern solar cell using silicon, with an efficiency of around 1%.
- 1954: Bell Labs develops the first practical solar cell with an efficiency of 4.6%, using a silicon p-n junction.
- 1958: The first solar-powered satellite, Vanguard 1, is launched.
- 1960s: Solar cells become more efficient (10-15%) and are used in space exploration.
- 1970s: Solar panels become more affordable and are used for terrestrial applications.
- 1980s: Thin-film solar cells are developed, reducing costs and increasing efficiency.
- 1990s: Multijunction solar cells are developed, achieving higher efficiencies (30-40%).
- 2000s: Solar energy becomes more mainstream, with large-scale solar power plants and residential installations.
- 2010s: Solar cell efficiencies continue to improve (40-46%), and costs decrease.
- Present day: Solar cells are used in various applications, from small devices to large power plants, with ongoing research to improve efficiency and reduce costs.
The history of solar cells is marked by continuous innovation, improving efficiency, and reducing costs, making solar energy a vital part of our transition to renewable energy sources.
Materials:
Solar cells are made from a variety of materials, each with its own advantages and disadvantages. Here are some common materials used in solar cell production:
- Silicon (Si): Most common material, accounting for 90% of solar cells. Crystalline silicon (c-Si) and amorphous silicon (a-Si) are used.
- Gallium Arsenide (GaAs): High-efficiency material, often used in multijunction solar cells.
- Cadmium Telluride (CdTe): Thin-film material, commonly used in commercial solar panels.
- Copper Indium Gallium Selenide (CIGS): Thin-film material, offers high efficiency and flexibility.
- Perovskite: New material with high efficiency and low production costs, still in development.
- Organic Photovoltaic (OPV): Made from organic materials, flexible and lightweight, still in development.
- Quantum Dot: Nanoparticles that increase efficiency, still in development.
- Germanium (Ge): Used in high-efficiency solar cells and multijunction solar cells.
- Indium Phosphide (InP): Used in high-efficiency solar cells and multijunction solar cells.
- Selenium (Se): Used in thin-film solar cells.
- Tandem Solar Cells: Combine multiple materials to increase efficiency.
- Bifacial Solar Cells: Use transparent backing sheet to absorb light from both sides.
Each material has its own advantages, disadvantages, and applications. Research continues to develop new materials and improve existing ones to increase efficiency, reduce costs, and enhance sustainability.
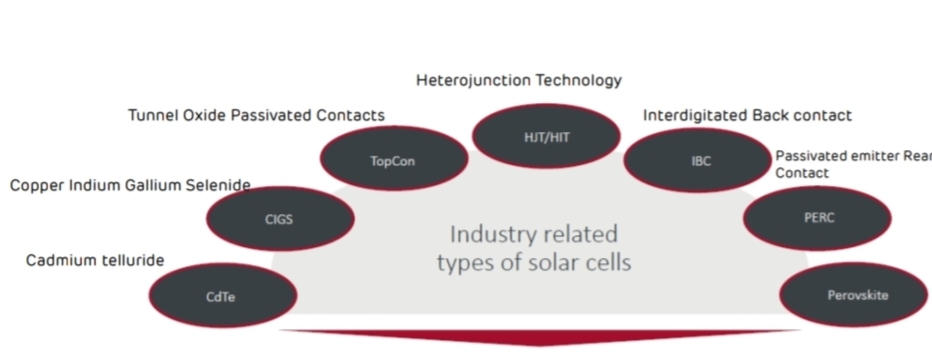
Structure:
A solar cell typically consists of the following layers:
- Top Contact: A thin metal grid or fingers that collect electrons and transfer them to an external circuit.
- p-type Semiconductor Layer (p-layer): A thin layer of positively charged (p-type) semiconductor material, usually silicon, which has an excess of holes (positive charge carriers).
- n-type Semiconductor Layer (n-layer): A thin layer of negatively charged (n-type) semiconductor material, usually silicon, which has an excess of electrons.
- p-n Junction: The region where the p-layer and n-layer meet, creating an electric field that separates electron-hole pairs.
- Antireflective Coating: A thin layer that reduces light reflection and increases light absorption.
- Back Contact: A metal layer that collects electrons and transfers them to an external circuit.
- Substrate: The base material, usually glass or plastic, that supports the solar cell.
- Encapsulant: A protective layer that seals the solar cell from the environment.
The p-n junction is the heart of the solar cell, where light absorption and electron-hole pair separation occur. The p-layer and n-layer are typically made from silicon, which is doped with impurities to create the p-type and n-type materials.
There are different types of solar cell structures, including:
- Monocrystalline Silicon Solar Cells: Made from single-crystal silicon.
- Polycrystalline Silicon Solar Cells: Made from multiple-crystal silicon.
- Thin-Film Solar Cells: Made from thin layers of photovoltaic material.
- Bifacial Solar Cells: Can absorb light from both the front and back sides.
- Perovskite Solar Cells: A newer type of solar cell with high efficiency and low production costs.
Each structure has its advantages and disadvantages, and research continues to improve their efficiency and affordability.
Working Principle:
A solar cell, also known as a photovoltaic (PV) cell, converts sunlight directly into electricity through a process known as photovoltaics. The working principle of a solar cell involves the following steps:
- Light Absorption: Solar cells are made from semiconducting materials like silicon. When sunlight hits a solar cell, it absorbs the energy from the light.
- Excitation of Electrons: The absorbed light energy excites the electrons in the semiconducting material, causing them to become energized and move freely.
- Creation of Electron-Hole Pairs: The energized electrons create pairs of electrons and holes (positive charge carriers). These pairs are formed when the electrons gain enough energy to break free from their atomic bonds.
- Separation of Electron-Hole Pairs: The electrons and holes are separated by an electric field in the solar cell, which is created by the p-n junction (a region where two types of semiconducting materials meet).
- Flow of Electrons: The separated electrons flow through the material, creating an electrical current. This current is collected and directed through an external circuit.
- Generation of Electricity: The flow of electrons through the circuit generates electricity, which can be used to power electrical devices or stored in batteries for later use.
In summary, solar cells convert sunlight into electricity by absorbing light, exciting electrons, creating electron-hole pairs, separating these pairs, and allowing the electrons to flow through a circuit to generate electricity.
Application:
Solar cells have numerous applications due to their ability to convert sunlight into electricity. Some of the most significant applications include:
- Renewable Energy Source: Solar cells provide a clean and sustainable source of energy, reducing reliance on fossil fuels and mitigating climate change.
- Powering Homes and Buildings: Solar panels composed of solar cells can power residential and commercial buildings, providing electricity for lighting, heating, and cooling.
- Solar Power Plants: Large-scale solar power plants generate electricity for the grid, supplying power to thousands of homes and businesses.
- Water Pumping and Irrigation: Solar cells power water pumps for irrigation, drinking water, and other agricultural needs.
- Electric Vehicles: Solar cells charge electric vehicles, reducing greenhouse gas emissions and dependence on fossil fuels.
- Street Lighting and Security Systems: Solar-powered streetlights and security systems provide reliable and energy-efficient lighting.
- Remote Power Generation: Solar cells provide electricity in remote areas where traditional power infrastructure is lacking.
- Consumer Electronics: Solar cells power small devices like calculators, watches, and chargers for smartphones and laptops.
- Space Exploration: Solar cells power satellites, spacecraft, and planetary rovers due to their high energy density and reliability.
- Agricultural Applications: Solar cells power farming equipment, greenhouses, and livestock management systems.
- Emergency Power Backup: Solar cells provide backup power during outages and natural disasters.
- Medical Devices: Solar cells power medical equipment, such as ventilators and blood pressure monitors, in remote or off-grid areas.
These applications showcase the versatility and potential of solar cells to transform the way we generate and use energy.
Manufacturing Process :
The manufacturing process of solar cells involves several stages:
- Purification of Silicon: Silicon is refined and purified to produce high-purity polysilicon.
- Ingot Formation: Polysilicon is melted and formed into a single-crystal ingot or a multi-crystal block.
- Wafer Production: The ingot is sliced into thin wafers, typically 150-200 μm thick.
- Texturization: The wafer surface is textured to increase light absorption.
- Diffusion: Dopants are diffused into the wafer to create the p-n junction.
- Deposition of Antireflective Coating: A thin layer is deposited to reduce light reflection.
- Metallization: Metal contacts are applied to the front and back of the cell.
- Screen Printing: Metal fingers and busbars are printed on the cell surface.
- Firing: The cell is heated to alloy the metal contacts and activate the p-n junction.
- Testing and Sorting: Cells are tested for efficiency and sorted according to their performance.
- Module Assembly: Cells are assembled into a module, sealed, and framed.
- Final Testing: The completed module is tested for performance and reliability.
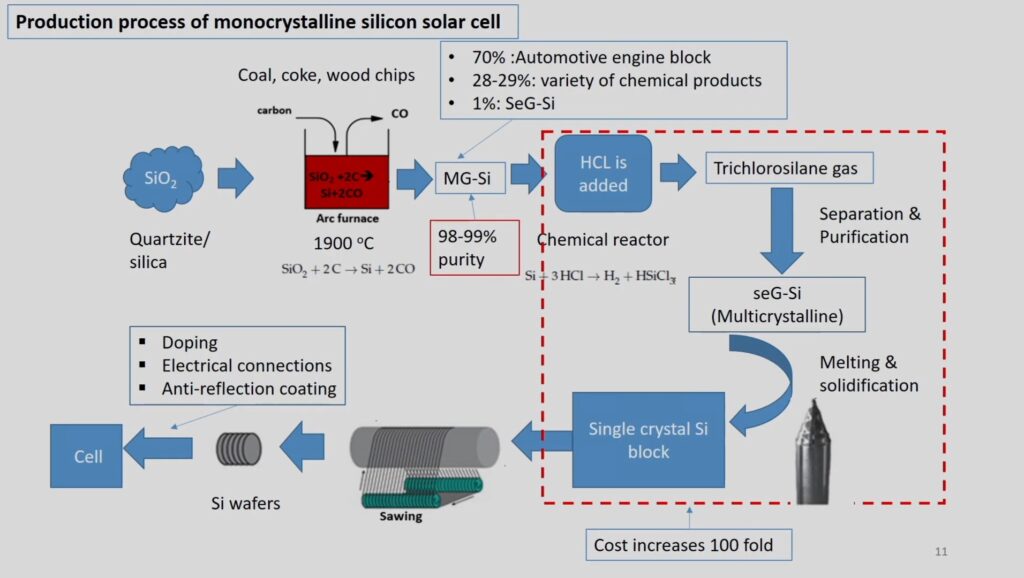
There are different manufacturing methods, including:
- Monocrystalline Silicon Process: Uses single-crystal silicon wafers.
- Polycrystalline Silicon Process: Uses multi-crystal silicon wafers.
- Thin-Film Process: Deposits photovoltaic material on a substrate.
- Czochralski Process: Grows single-crystal silicon ingots.
- Float Zone Process: Produces high-purity silicon wafers.
Each method has its advantages and disadvantages, and manufacturers continue to improve efficiency and reduce costs.
Research and industrial production :
Research and industrial production of solar cells are ongoing, with advancements in efficiency, cost reduction, and new technologies. Some current research areas include:
- Bifacial Solar Cells (TopCon): Absorb light from both sides, increasing efficiency.
- Perovskite Solar Cells: New material with high efficiency and low production costs.
- Multi-Junction Solar Cells: Stack multiple layers to increase efficiency.
- Thin-Film Solar Cells: Reduce material usage and costs.
- Quantum Dot Solar Cells: Use nanoparticles to increase efficiency.
- Concentrated Photovoltaic (CPV) Cells: Use lenses or mirrors to focus sunlight.
- Silicon Heterojunction Technology: Improve efficiency and reduce costs.
- Tandem Solar Cells: Stack different materials to increase efficiency.
Industrial production involves:
- Scaling up manufacturing processes.
- Improving material quality and consistency.
- Reducing production costs.
- Increasing automation and efficiency.
- Developing new manufacturing technologies.
Some notable companies involved in solar cell research and production include:
- SunPower
- Tesla
- Panasonic
- LG Solar
- Trina Solar
- Jinko Solar
- JA Solar
- Canadian Solar
- Hanwha Q CELLS
- First Solar
Research institutions and organizations also play a crucial role in advancing solar cell technology, such as:
- National Renewable Energy Laboratory (NREL)
- Fraunhofer Institute for Solar Energy Systems
- University of California, Berkeley
- Massachusetts Institute of Technology (MIT)
- International Energy Agency (IEA) Photovoltaic Power Systems Programme
The solar industry continues to evolve, with innovations and economies of scale driving growth and adoption.
Declining costs and exponential growth:
The cost of solar cells has declined dramatically over the years, leading to exponential growth in the adoption of solar energy. Here are some key factors contributing to this trend:
- Economies of scale: As demand increases, manufacturers can produce larger quantities, reducing costs per unit.
- Technological advancements: Improvements in efficiency, materials, and manufacturing processes have reduced costs.
- Increased competition: More companies entering the market have driven prices down.
- Government incentives and policies: Support for renewable energy has encouraged adoption and reduced costs.
- Learning curve: As the industry grows, manufacturers learn to optimize production, reducing costs.
The results are impressive:
- Cost reduction: The cost of solar cells has fallen by over 70% in the last decade.
- Exponential growth: Solar energy capacity has grown exponentially, with a compound annual growth rate (CAGR) of over 20%.
- Increased adoption: Solar energy now accounts for a significant share of global power generation.
- Grid parity: Solar energy has reached grid parity in many regions, making it competitive with fossil fuels.
- Record installations: New records are set regularly for solar installations, driving growth and reducing costs further.
Some key statistics illustrating this trend include:
- 2010: Solar cell cost – $1.80/Watt, Global solar capacity – 40 GW
- 2020: Solar cell cost – $0.20/Watt, Global solar capacity – 720 GW
- 2030 projections: Solar cell cost – $0.10/Watt, Global solar capacity – 3,000 GW (IEA forecast)
The declining costs and exponential growth of solar cells have made solar energy a vital part of the global transition to renewable energy sources.
Efficiency:
Solar cell efficiency refers to the percentage of sunlight that is converted into electrical energy. The efficiency of solar cells has increased over the years due to advancements in technology and materials. Here are some key efficiency milestones:
- 1954: First commercial solar cell – 4.6% efficient
- 1960s: Solar cells reach 10-15% efficiency
- 1980s: Thin-film solar cells emerge with 10-15% efficiency
- 1990s: Multijunction solar cells reach 30-40% efficiency
- 2010s: Solar cells with efficiencies above 20% become common
- 2020s: Bifacial solar cells reach efficiencies above 25%
- Lab records: Solar cells with efficiencies above 46% (multi-junction) and 29% (single-junction)
Commercial solar panels typically have efficiencies between 15-20%, while high-efficiency panels can reach up to 24%. Bifacial solar cells, which absorb light from both sides, can reach efficiencies above 25%.
Factors affecting solar cell efficiency include:
- Material quality
- Design and architecture
- Manufacturing process
- Temperature
- Light intensity
Increasing efficiency is crucial for:
- Reducing material usage
- Lowering costs
- Increasing energy output
- Improving sustainability
Researchers continue to push the boundaries of solar cell efficiency, exploring new materials and technologies to achieve even higher efficiencies.
Certification:
Here is a list of certification bodies for solar cells:
- UL (Underwriters Laboratories): Tests and certifies solar cells to ensure compliance with safety standards.
- ETL (Intertek): Certifies solar cells to North American safety standards.
- IEC (International Electrotechnical Commission): Develops and publishes international standards for solar cells.
- ISO (International Organization for Standardization): Certifies solar cells to international quality and environmental management standards.
- TÜV Rheinland: Certifies solar cells to international safety and performance standards.
- SGS: Inspects and certifies solar cells to ensure compliance with international standards.
- CSA Group: Certifies solar cells to North American safety standards.
- DEKRA: Certifies solar cells to international safety and performance standards.
- Intertek: Certifies solar cells to international safety and performance standards.
- DNV GL: Certifies solar cells to international safety and performance standards.
- Bureau Veritas: Certifies solar cells to international safety and performance standards.
- CEC (California Energy Commission): Certifies solar cells to California’s safety and performance standards.
- FSEC (Florida Solar Energy Center): Certifies solar cells to Florida’s safety and performance standards.
These certification bodies ensure that solar cells meet rigorous standards for quality, safety, and performance, giving consumers confidence in their solar energy systems.
Disposal:
The disposal of solar cells, also known as photovoltaic (PV) modules, is an important consideration as the industry grows and older modules reach the end of their life. Here are some ways to dispose of solar cells:
- Recycling: Solar cells can be recycled to recover valuable materials like silicon, aluminum, and copper.
- Proper disposal: Solar cells can be disposed of in landfills, but they must be handled and stored properly to prevent environmental harm.
- Reusing: Solar cells can be reused in other applications, such as building-integrated photovoltaics (BIPV).
- Repurposing: Solar cells can be repurposed for other uses, like charging small devices or powering outdoor lighting.
- Donation: Still-functional solar cells can be donated to organizations or communities in need.
- Manufacturer take-back programs: Some manufacturers offer take-back programs for old solar cells.
- Solar cell recycling facilities: Specialized facilities can recycle solar cells to recover materials.
- Research and development: Old solar cells can be used in research and development to improve recycling methods and develop new technologies.
It’s essential to handle solar cells with care during disposal to prevent:
- Toxic material release
- Environmental contamination
- Waste accumulation
The solar industry is working to develop more sustainable and responsible end-of-life management practices for solar cells.
Recycling:
Solar cell recycling is the process of recovering valuable materials from old or damaged solar panels. The recycling process typically involves:
- Disassembly: Breaking down the solar panel into its components, such as glass, frames, and cells.
- Cell separation: Separating the solar cells from the module.
- Material sorting: Sorting materials like silicon, aluminum, copper, and glass.
- Shredding: Shredding the materials into smaller pieces.
- Melting: Melting the materials to produce raw materials.
- Purification: Purifying the materials to produce high-quality raw materials.
Recyclable materials from solar cells include:
- Silicon: Used to produce new solar cells or semiconductors.
- Aluminum: Used in transportation, construction, and packaging.
- Copper: Used in electrical applications, construction, and consumer goods.
- Glass: Used in construction, packaging, and fiberglass production.
- Silver: Used in electronics, solar panels, and other applications.
Benefits of solar cell recycling:
- Conservation of resources
- Reduction of waste
- Decreased environmental impact
- Cost savings
- Job creation
Challenges in solar cell recycling:
- Lack of infrastructure
- High costs
- Technical difficulties
- Limited market demand
- Regulatory frameworks
As the solar industry grows, recycling will play a crucial role in sustaining its environmental benefits and reducing waste.